The term nitrification refers to the conversion of ammonium to nitrate (pathway 3–4). This is brought about by the nitrifying bacteria, which are specialised to gain their energy by oxidising ammonium, while using CO₂ as their source of carbon to synthesise organic compounds.
Organisms of this sort are termed chemoautotrophs – they gain their energy by chemical oxidations (chemo-) and they are autotrophs (self-feeders) because they do not depend on pre-formed organic matter.
In principle, the oxidation of ammonium by these bacteria is no different from the way in which humans gain energy by oxidising sugars. Their use of CO₂ to produce organic matter is no different in principle from the behaviour of plants.
The nitrifying bacteria are found in most soils and waters of moderate pH, but are not active in highly acidic soils.
They almost always are found as mixed-species communities (termed consortia) because some of them – e.g., Nitrosomonas species are specialised to convert ammonium to nitrite (NO₂⁻) while others e.g., Nitrobacter species convert nitrite to nitrate (NO₃⁻).
In fact, the accumulation of nitrite inhibits Nitrosomonas, so it depends on Nitrobacter to convert this to nitrate, whereas Nitrobacter depends on Nitrosomonas to generate nitrite.
The nitrifying bacteria have some important environmental consequences, because they are so common that most of the ammonium in oxygenated soil or natural waters is readily converted to nitrate.
Most plants and microorganisms can take up either nitrate or ammonium (arrows marked “1” in the diagram). However, the process of nitrification has some undesirable consequences.
The ammonium ion (NH₄⁺) has a positive charge and so is readily adsorbed onto the negatively charged clay colloids and soil organic matter, preventing it from being washed out of the soil by rainfall.
In contrast, the negatively charged nitrate ion is not held on soil particles and so can be washed down the soil profile – the process termed leaching (arrow marked 7 in the diagram). In this way, valuable nitrogen can be lost from the soil, reducing the soil fertility.
The nitrates can then accumulate in groundwater, and ultimately in drinking water. There are strict regulations governing the amount of nitrate that can be present in drinking water, because nitrates can be reduced to highly reactive nitrites by microorganisms in the anaerobic conditions of the gut.
Raed Also: Blackleg (cruciferous plants): Description, Damages Caused, Control and Preventive Measures
Nitrites are absorbed from the gut and bind to haemoglobin, reducing its oxygen-carrying capacity. In young babies this can lead to respiratory distress – the condition known as “blue baby syndrome”. Nitrite in the gut also can react with amino compounds, forming highly carcinogenic nitrosamines.
Microbial Conversion of Nitrate into Gaseous Forms
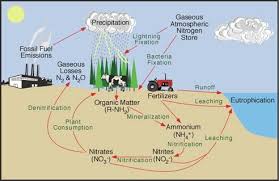
Denitrification refers to the process in which nitrate is converted to gaseous compounds (nitric oxide, nitrous oxide and N₂) by microorganisms.
The sequence usually involves the production of nitrite (NO₂⁻) as an intermediate step as shown as “5” in the diagram above. Several types of bacteria perform this conversion when growing on organic matter in anaerobic conditions.
Because of the lack of oxygen for normal aerobic respiration, they use nitrate in place of oxygen as the terminal electron acceptor. This is termed anaerobic respiration and can be illustrated as follows:
In aerobic respiration (as in humans), organic molecules are oxidised to obtain energy, while oxygen is reduced to water:
C₆H₁₂O₆ + 6 O₂ = 6 CO₂ + 6 H₂O + energy
In the absence of oxygen, any reducible substance such as nitrate (NO₃⁻) could serve the same role and be reduced to nitrite, nitric oxide, nitrous oxide or N₂.
Thus, the conditions in which denitrifying organisms are found are characterised by
- a supply of oxidisable organic matter, and
- absence of oxygen but availability of reducible nitrogen sources. A mixture of gaseous nitrogen products is often produced because of the stepwise use of nitrate, nitrite, nitric oxide and nitrous oxide as electron acceptors in anaerobic respiration.
The common denitrifying bacteria include several species of Pseudomonas, Alkaligenes and Bacillus. Their activities result in substantial losses of nitrogen into the atmosphere, roughly balancing the amount of nitrogen fixation that occurs each year.
Nitrogen-Fixing Role of the Legume Family in Agriculture
Plants that contribute to nitrogen fixation include the legume family – Fabaceae – with taxa such as clovers, soybeans, alfalfa, lupines, peanuts, and rooibos. They contain symbiotic bacteria called Rhizobia within nodules in their root systems, producing nitrogen compounds that help the plant to grow and compete with other plants.
When the plant dies, the fixed nitrogen is released, making it available to other plants and this helps to fertilize the soil. The great majority of legumes have this association, but a few genera (e.g., Styphnolobium) do not.
In many traditional and organic farming practices, fields are rotated through various types of crops, which usually includes one consisting mainly or entirely of clover or buckwheat (non-legume family Polygonaceae), which are often referred to as “green manure.” Inga alley farming relies on the leguminous genus Inga, a small tropical, tough-leaved, nitrogen-fixing tree.
Non-Leguminous Nitrogen-Fixing Plants
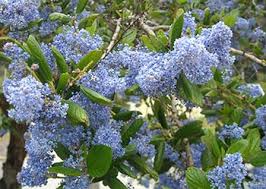
Although the majority of plants able to form nitrogen-fixing root nodules are in the legume family Fabaceae, there are a few exceptions:
i. Parasponia, a tropical Celtidaceae also able to interact with *Rhizobia* and form nitrogen-fixing nodules
ii. Actinorhizal plants such as alder and bayberry, that can also form nitrogen-fixing nodules, thanks to a symbiotic association with Frankia bacteria
These plants belong to 25 genera distributed among 8 plant families. The ability to fix nitrogen is far from universally present in these families. For instance, of 122 genera in the Rosaceae, only 4 genera are capable of fixing nitrogen.
All these families belong to the orders Cucurbitales, Fagales, and Rosales, which together with the Fabales form a clade of eurosids. In this clade, Fabales were the first lineage to branch off; thus, the ability to fix nitrogen may be plesiomorphic and subsequently lost in most descendants of the original nitrogen-fixing plant.
However, it may be that the basic genetic and physiological requirements were present in an incipient state in the last common ancestors of all these plants, but only evolved to full function in some of them:
i. Coriariaceae: Coriaria
ii. Datiscaceae: Datisca
iii. Elaeagnaceae*: *Elaeagnus* (silverberries), Hippophae (sea-buckthorns), Shepherdia (buffaloberries)
Other Nitrogen-Fixing Symbiotic Associations
There are also several nitrogen-fixing symbiotic associations that involve cyanobacteria (such as Nostoc):
- Some lichens such as Lobaria and Peltigera
- Mosquito fern (Azolla species)
- Cycads
- Gunnera
Raed Also: Blackleg (cruciferous plants): Description, Damages Caused, Control and Preventive Measures
Chemical Nitrogen Fixation and Its Application in Agriculture
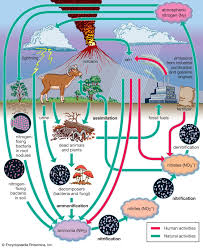
1. The Haber Process and Fertiliser Production
Nitrogen can also be artificially fixed as ammonia for use in fertilizers, explosives, or in other products. The most common method is the Haber process.
Artificial fertilizer production is now the largest source of human-produced fixed nitrogen in the Earth’s ecosystem. The Haber process requires high pressures (around 200 atm) and high temperatures (at least 400 °C), routine conditions for industrial catalysis. This highly efficient process uses natural gas as a hydrogen source and air as a nitrogen source.
2. Dinitrogen Complexes and Synthetic Applications
Much research has been conducted on the discovery of catalysts for nitrogen fixation, often with the goal of reducing the energy required for this conversion. However, such research has thus far failed to even approach the efficiency and ease of the Haber process.
Many compounds react with atmospheric nitrogen under ambient conditions. For example, lithium metal converts to lithium nitride under an atmosphere of nitrogen. Treatment of the resulting nitride gives ammonia.
The first dinitrogen complex was reported in 1965 based on ammonia coordinated to ruthenium ([Ru(NH₃)₅(N₂)]²⁺). Research in chemical fixation from then on focused on transition metal complexes.
Since then, a large number of transition metal compounds that contain dinitrogen as a ligand have been discovered.
The dinitrogen ligand can either be bound to a single metal or bridge two (or more) metals. The coordination chemistry of dinitrogen is complex and currently under intense investigation. This research may lead to new ways of using dinitrogen in synthesis and on an industrial scale.
3. Ambient Nitrogen Reduction Techniques
Catalytic chemical nitrogen fixation at temperatures considerably lower than the Haber process is an ongoing scientific endeavor. Nitrogen was successfully converted to ammonia and hydrazine by Alexander E. Shilov in 1970. The first example of homolytic cleavage of dinitrogen under mild conditions was published in 1995.
Two equivalents of a molybdenum complex reacted with one equivalent of dinitrogen, creating a triple bonded MoN complex. Since then, this triple bonded complex has been used to make nitriles.
The first catalytic system converting nitrogen to ammonia at room temperature and pressure was discovered in 2003 and is based on another molybdenum compound, a proton source, and a strong reducing agent.
However, this catalytic reduction fixates only a few nitrogen molecules. In 2011, Arashiba et al. reported another system with a catalyst again based on molybdenum but with a diphosphorus pincer ligand.
Do you have any questions, suggestions, or contributions? If so, please feel free to use the comment box below to share your thoughts. We also encourage you to kindly share this information with others who might benefit from it. Since we can’t reach everyone at once, we truly appreciate your help in spreading the word. Thank you so much for your support and for sharing!