Fish perform all their bodily functions in water. Because fish are totally dependent upon water to breathe, feed, grow, excrete wastes, maintain a salt balance, and reproduce, understanding the physical and chemical qualities of water is critical to successful aquaculture.
To a great extent, water determines the success or failure of an aquaculture operation. This article is designed to help students understand the properties of water as they affect fish culture.
Physical Characteristics of Water in Aquaculture
Water can hold large amounts of heat with a relatively small change in temperature. This heat capacity has far-reaching implications. It permits a body of water to act as a buffer against wide fluctuations in temperature. The larger the body of water, the slower the rate of temperature change. Furthermore, aquatic organisms take on the temperature of their environment and cannot tolerate rapid changes in temperature.
Water has very unique density qualities. Most liquids become denser as they become cooler. Water, however, gets denser as it cools until it reaches a temperature of approximately 4°C. As it cools below this point, it becomes lighter until it freezes at 0°C. As ice develops, water increases in volume by 11 percent. The increase in volume allows ice to float rather than sink, a characteristic that prevents ponds from freezing solid.
Read Also: How to Extract and Package Snail Slime (Snail filtrate) for Commercial Use
Water Balance in Fish: Freshwater vs. Marine Species
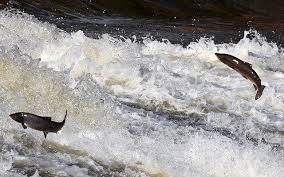
The elimination of most nitrogen waste products in land animals is performed through the kidneys. In contrast, fish rely heavily on their gills for this function, excreting primarily ammonia. A fish’s gills are permeable to water and salts. In the ocean, the salinity of water is more concentrated than that of the fish’s body fluids.
In this environment, water is drawn out, but salts tend to diffuse inward. Hence, marine fishes drink large amounts of seawater and excrete small amounts of highly salt-concentrated urine.
In freshwater fish, water regulation is the reverse of marine species. Salt is constantly being lost through the gills, and large amounts of water enter through the fish’s skin and gills. This is because the salt concentration in a fish (approximately 0.5 percent) is higher than the salt concentration of the water in which it lives.
Because the fish’s body is constantly struggling to prevent the “diffusion” of water into its body, large amounts of water are excreted by the kidneys. As a result, the salt concentration of the urine is very low. By understanding the need to maintain a water balance in freshwater fish, one can understand why using salt during transport is beneficial to fish.
Sources of Water for Aquaculture
Water is always a limiting factor in commercial fish production. Many of the negative chemical and environmental factors associated with most operations have their origins in the source of water selected. Final site selection has to be made based on both the quality and quantity of water available.
The most common sources of water used for aquaculture are wells, springs, rivers, lakes, groundwater, rainfall, and municipal water. Of the sources mentioned, rainfall, wells, and springs are considered to consistently be of high quality.
Key Physical Factors Affecting Water in Aquaculture
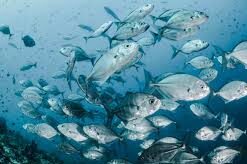
1. Temperature in Aquaculture Systems
After oxygen, water temperature may be the single most important factor affecting the welfare of fish. Fish are cold-blooded organisms and assume approximately the same temperature as their surroundings.
The temperature of the water affects the activity, behavior, feeding, growth, and reproduction of all fishes. Metabolic rates in fish double for each 8°C rise in temperature. Channel catfish and tilapia are examples of warm-water species. Their temperature range for growth is between 24-32°C. A temperature of 29°C for catfish and 31°C for tilapia is considered optimum.
Walleye and yellow perch are examples of cool-water species. Ranges for optimum growth fall between 16 and 29°C. Temperature at the upper end of this range is considered best for maximum growth for most cool-water species.
Coldwater species include all species of salmon and trout. The most commonly cultured coldwater species in the Midwest is rainbow trout, whose optimal temperature range for growth is 9-18°C. Ideally, species selection should be based in part on the temperature of the water supply. Any attempt to match a fish with less than ideal temperatures will involve energy expenditures for heating or cooling.
Temperature also determines the amount of dissolved gases (oxygen, carbon dioxide, nitrogen, etc.) in the water. The cooler the water, the more soluble the gas. Temperature plays a major role in the physical process called thermal stratification.
As a result of the uniqueness of water in terms of density and high heat capacity, nutrients, dissolved gases, and fish wastes are evenly mixed throughout the pond. As the days become warmer, the surface water becomes warmer and lighter while the cooler, denser water forms a layer underneath.
Circulation of the colder bottom water is prevented because of the different densities between the two layers of water. Dissolved oxygen levels decrease in the bottom layer since photosynthesis and contact with the air are reduced. The already low oxygen levels are further reduced through the decomposition of waste products, which settle to the pond bottom. Localized dissolved oxygen depletion poses a very real problem to the fish farmer.
2. Suspended Solids in Aquaculture Water
Suspended solids are associated with plankton, fish wastes, uneaten fish feeds, or clay particles suspended in the water. Suspended solids are large particles that usually settle out of standing water over time. Large clay particles are an exception, while small clay particles are kept in suspension due to the negative electrical charges associated with them.
3. Plankton and Its Role in Water Quality
Turbidity caused by phytoplankton (microscopic plants) and zooplankton (microscopic animals) is not directly harmful to fish. Phytoplankton (green algae) not only produces oxygen but also provides a food source for zooplankton and filter-feeding fish/shellfish.
Phytoplankton also uses ammonia produced by fish as a nutrient source. Zooplankton is a very important food source for fry and fingerlings such as hybrid striped bass and yellow perch. However, excessive amounts of algae can lead to increased rates of respiration during the night, thereby consuming extra oxygen.
Excessive phytoplankton buildup or “blooms” that subsequently die will also consume extra oxygen. Any wide disparity between day and night oxygen levels can lead to dangerously low oxygen concentrations.
Fish Wastes and Water Quality
Suspended fish wastes are a serious concern for water recirculating culture systems. Large amounts of suspended and settleable solids are produced during fish production. Therefore, fish waste particles can be a major source of poor water quality since they may contain up to 70 percent of the nitrogen load in the system.
These wastes not only irritate the fish’s gills but can also cause several problems for the biological filter. The particulate waste can clog the biological filter, causing the nitrifying bacteria to die from a lack of oxygen. Particulate waste can also promote the growth of bacteria that produce rather than consume ammonia.
Clay Turbidity in Aquaculture Systems
Most clay turbidity problems are the result of exposed soil on the pond levee, exposed watershed, or feeding on bottom-dwelling organisms. In natural bodies of water, turbidity values seldom exceed critical levels.
Even muddy-looking ponds rarely have concentrations greater than 2,000 ppm. Turbidity caused by clay or soil particles, however, can restrict light penetration and limit photosynthesis. Sedimentation of soil particles may also smother fish eggs and destroy beneficial communities of bottom organisms.
Removal of clay turbidity can be accomplished by adding materials that attach to the negative charges of the clay particles, forming particles heavy enough to settle to the bottom. Common remedies for clay turbidity are 7-10 square bales of hay per surface acre or 300-500 pounds of gypsum per surface acre. Gypsum application may be repeated at two-week intervals if the pond is still not clear.
Chemical Factors Affecting Water Quality in Aquaculture
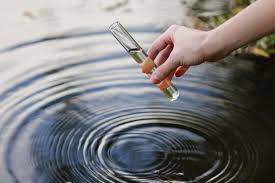
1. Photosynthesis in Aquaculture Systems
Photosynthesis is one of the most important biological activities in standing pond aquaculture. Many water quality parameters such as dissolved oxygen, carbon dioxide, pH cycles, and nitrogenous waste products are regulated by the photosynthetic reaction in phytoplankton.
It can be simply put as the process by which phytoplankton uses sunlight to convert carbon dioxide into a food source and release oxygen as a by-product. This process can be summarized as the equation below:
CH2O (food) + O2 ↔ CO2 + H2O + energy ↑
In addition to supplying oxygen in fish ponds, photosynthesis also removes several forms of nitrogenous wastes, such as ammonia, nitrates, and urea. The phytoplanktonic plant pigments involved in this chemical reaction are referred to as chlorophyll.
Because the photosynthetic process is driven by sunlight, the greatest concentrations of oxygen occur when the sun is highest on the horizon, while phytoplankton primarily respire at night when photosynthesis ceases.
Respiration is the reverse of photosynthesis in that oxygen is used by phytoplankton to convert food to energy, and carbon dioxide is released as a by-product. Phytoplankton respiration also occurs during the day, but the fish farmer is fortunate since there is usually a surplus of oxygen produced to compensate for the loss.
2. Dissolved Gases in Aquaculture Water
Dissolved gases are gases that are in water solution. An example of a gas dissolved in water is soda water, which has large quantities of dissolved carbon dioxide. Concentrations are measured in parts per million (ppm) or milligrams per liter (mg/L), both units of measure are the same. (One ppm or mg/L is the same as one pound added to 999,999 pounds to make 1,000,000 pounds).
Oxygen: The Most Critical Chemical Parameter
Dissolved oxygen (DO) is by far the most important chemical parameter in water for fish production. Low DO levels are responsible for more fish kills, either directly or indirectly, than all other problems combined.
Like humans, fish require oxygen for respiration, and the amount of oxygen consumed by the fish is a function of its size, feeding rate, activity level, and temperature. Small fish consume more DO than large fish because of their higher metabolic rate.
Fish farmers, in an attempt to maximize production, stock greater amounts of fish in a given body of water than found in nature, especially when using surface ponds or concrete tanks.
.
Therefore, it may be necessary to supply supplemental aeration to maintain adequate levels of dissolved oxygen. Whereas in Recirculatory Aquaculture Systems (RAS), the farmer must supply 100 percent of the oxygen needed for the fish and beneficial nitrifying bacteria.
To obtain good growth, fish must be cultured at optimum levels of DO. A good rule of thumb is to maintain DO levels at saturation or at least 5 ppm. DO levels less than 5 ppm can place undue stress on the fish, and levels less than 2 ppm will result in death. Some warm-water fish species such as tilapia and carp are better adapted to withstand occasional low DO levels, while most coldwater species cannot.
Fish are not the only consumers of dissolved oxygen in aquaculture systems; bacteria, phytoplankton, and zooplankton consume large quantities of oxygen as well. Decomposition of organic materials (algae, bacteria, and fish wastes) is the single greatest consumer of oxygen in aquaculture systems.
Problems encountered from water recirculating systems usually stem from excessive ammonia production in fish wastes. Consumption of oxygen by nitrifying bacteria that break down toxic ammonia to non-toxic forms depends on the amount of ammonia entering the system.
Oxygen enters the water primarily through direct diffusion at the air-water interface and through photosynthesis. Direct diffusion is relatively insignificant unless there is considerable wind and wave action. Several forms of mechanical aeration are available to the fish farmer. Mechanical aeration can also increase DO levels.
Because of the lack of photosynthesis in indoor water recirculating systems, mechanical means of aeration is the only alternative for supplying oxygen to aquatic animals cultured in the systems. Oxygen depletions can be calculated, but predictions can be misleading and should never be substituted for actual measurements. Categories of mechanical aerators include:
- Paddlewheels
- Agitators
- Vertical sprayers
- Impellers
- Airlift pumps
- Venturi pumps
- Liquid oxygen injection
- Air diffusers
Carbon Dioxide in Aquaculture Systems
Carbon dioxide (CO2) is commonly found in water from photosynthesis or from water sources originating from limestone-bearing rock. Fish can tolerate concentrations of 10 ppm provided dissolved oxygen concentrations are high. Water supporting good fish populations normally contains less than 5 ppm of free carbon dioxide.
In water used for intensive pond fish culture, carbon dioxide levels may fluctuate from 0 ppm in the afternoon to 5-15 ppm at daybreak. In recirculating aquaculture systems, carbon dioxide levels may regularly exceed 20 ppm. Excessively high levels of carbon dioxide (greater than 20 ppm) may interfere with the oxygen utilization by the fish.
There are two common ways to remove free carbon dioxide. First, with well or spring water from limestone-bearing rocks, aeration can blow off excess gas. The second option is to add some type of carbonate buffering material such as calcium carbonate (CaCO3) or sodium bicarbonate (Na2CO3).
These additions require calculating the exact amount of the material needed for that purpose, as it will initially remove all free carbon dioxide and store it in reserve as bicarbonate and carbonate buffers.
Nitrogen and Gas Supersaturation
Dissolved gases, especially nitrogen, are usually measured in terms of “percent saturation.” Any value greater than the amount of gas the water normally holds at a given temperature constitutes supersaturation. A gas supersaturation level above 110% is usually considered problematic.
Gas bubble disease, which may vary in signs, is a symptom of gas supersaturation. Bubbles may reach the heart or brain, and fish may die without any visible external signs. Other symptoms may include bubbles just under the surface of the skin, in the eyes, or between the fin rays. Treatment of gas bubble disease involves sufficient aeration to decrease the gas concentration to saturation or below.
Ammonia in Aquaculture Systems
Fish excrete ammonia and lesser amounts of urea into the water as wastes. Two forms of ammonia occur in aquaculture systems: ionized and un-ionized. The un-ionized form of ammonia (NH3) is extremely toxic, causing brown-blood disease in fish, while the ionized form (NH4+) is not.
Both forms are grouped together as “total ammonia.” Through biological processes called nitrification, toxic ammonia can be degraded to harmless nitrates according to the following equation:
NH3 + 1½ O2 → NO2- + 1½ O2 → NO3-
In natural waters, such as lakes, ammonia may never reach dangerously high levels because of the low densities of fish. However, the fish farmer must maintain high densities of fish in ponds and, therefore, runs the risk of ammonia toxicity, which is mostly favored by a rise in temperature and high pH.
pH Levels in Aquaculture
The quantity of hydrogen ions (H+) in water will determine if it is acidic or basic. The scale for measuring the degree of acidity is called the pH scale, which ranges from 1 to 14. A value of 7 is considered neutral, neither acidic nor basic; values below 7 are considered acidic, while values above 7 are considered basic. The acceptable range for fish culture is between 6.5 and 9.0.
Alkalinity in Aquaculture Water
Alkalinity is the capacity of water to neutralize acids without an increase in pH. This parameter is a measure of bases, bicarbonates (HCO3-), carbonates (CO3–), and, in rare instances, hydroxide (OH-). Total alkalinity is the sum of the carbonates and bicarbonates alkalinities.
The carbonate buffering system is important to the fish farmer regardless of the method of production, as it helps to minimize daily pH fluctuations.
In recirculating systems where photosynthesis is practically non-existent, a good buffering capacity can prevent excessive buildups of carbon dioxide and lethal decreases in pH. It is recommended that fish farmers maintain total alkalinity values of at least 20 ppm for catfish production.
Water Hardness in Aquaculture
Water hardness is similar to alkalinity but represents different measurements. Hardness is chiefly a measure of calcium and magnesium, but other ions such as aluminum, iron, manganese, strontium, zinc, and hydrogen ions are also included.
When the hardness level is equal to the combined carbonate and bicarbonate alkalinity, it is referred to as carbonate hardness. Hardness values greater than the sum of the carbonate and bicarbonate alkalinity are referred to as non-carbonated hardness. Hardness values of at least 20 ppm should be maintained for optimum growth of fish and other aquatic organisms. Low hardness values can be increased with the addition of ground agricultural lime.
Other Metals and Gases in Aquaculture
Other metals such as iron and sodium, and gases such as hydrogen sulfide, may sometimes present special problems to the fish farmer. Most complications arising from these can be prevented by properly pre-treating the water prior to adding it to ponds or tanks.
The range of treatments may be as simple as aeration, which removes hydrogen sulfide gas, to the expensive use of iron removal units. Normally, iron will precipitate out of solution upon exposure to adequate concentrations of oxygen at a pH greater than 7.0.
The Importance of Water Quality in Aquaculture
Phytoplankton are microscopic photosynthetic organisms found suspended in water. Phytoplankton are found in most bodies of fresh and saltwater, including channel catfish ponds. Soon after a fish pond is dug and filled, samples of different species of phytoplankton can be found.
These new ponds can be seeded with phytoplankton through such sources as wading birds, turtles, and wind-blown spores. Many species of phytoplankton will reach a new pond, but only certain ones will survive and flourish.
The species that colonizes the new pond depends upon the suitability of the environment for growth and, to some extent, chance. It is not uncommon for ponds that are constructed side-by-side with similar soil types and the same water sources to contain very different populations of phytoplankton.
1. Eutrophication in Aquaculture Systems
Eutrophication is the aging process, ending in “death,” that takes place in a pond as it begins to be overgrown with the characteristics of a bog or lagoon.
A dead pond is unable to support aquatic life and so should be guarded against for a successful fish farming business. The following descriptions summarize the rate and level of eutrophication attained by a particular water body, based on the physical and chemical characteristics.
2. Oligotrophic (Very Healthy)
This indicates a very healthy pond or water body. Such a pond is stable with good clarity and water quality and is aging at a slower than normal rate.
The ecosystem is balanced and able to convert all nutrients to all levels of the food chain. This situation supports fish life, and production is enhanced through maximum utilization of the entire food chain.
3. Mesotrophic (Moderately Healthy)
This pond condition may need aeration and possibly enzymes too. This indicates that the pond is aging progressively at a normal rate with good water quality. Benthic organisms are converting and oxidizing the organics falling to the bottom on demand or slightly slower than demand.
4. Eutrophic (Unhealthy)
This shows that aeration and enzymes are necessary. The implication of this is that the water quality is eroding at an unhealthy accelerated pace. Efforts must be made to reverse the continued aging process.
Organic matter is accumulating, undecomposed and unconverted to the benthic layer, which is strangling the lake and all organisms that inhabit it. The low oxygen condition will result in periodic fish kills if un-aerated.
5. Hyper Eutrophic (Severely Degraded)
It is a must to have aeration and enzymes in this situation. Aging is accelerated beyond normal due to pollution and other influences. The pond will not be able to sustain a balanced ecosystem of life for very long.
Immediate and long-term plans must be made to retard the eutrophication process. Stratification is clearly evident, and bottom-dwelling organisms and bacteria are unable to convert and oxidize the rapid deposition of organic sludge.
Also evident are excessive weed and algae growth, low dissolved oxygen, and fish kills or asphyxiation as a result of high levels of nutrients in the water body. In this regard, phosphorus measured in open water is the most accurate indicator of primary productivity.
6. Phytoplankton Management in Aquaculture
To date, there is no effective way to manage a phytoplankton bloom other than reducing nutrient input in the form of feeds. This will limit the nutrients (phosphorus, nitrogen) available for growth and reproduction of the phytoplankton community and will result in a moderate phytoplankton density.
In a catfish pond, this is around 40 to 50 pounds of feed/acre/day. (This is not an economical option for most catfish producers.) Other methods have been used to try to control phytoplankton densities but have been completely ineffective.
Studies have shown that the use of copper sulfate (CuSO4) to selectively thin the bloom does much more harm than good. Thinning the phytoplankton bloom in this manner results in very poor water quality and overall reduced fish production.
The use of dyes to shade out dense phytoplankton blooms has been studied. Dyes probably result in poorer water quality throughout the growing season. Dyes may also select for undesirable blue-green algae in treated ponds.
Beneficial Practices to Improve Water Quality in Aquaculture
Beneficial (or best) management practices (BMPs) is the term used to describe a practice or system of practices designed to minimize the impact of agricultural activities on natural resources while at the same time maintaining the economic viability of the agricultural industry. To ensure a supply of good quality water for aquaculture, agricultural purposes, and domestic use, it is necessary to consider BMPs at three levels:
1. Watershed Management: To protect both surface and groundwater sources.
2. Water Source Management: Such as a dugout, river, or groundwater aquifer.
- Water Treatment for Use
Watershed Management Techniques
Watershed management techniques offer the first water treatment strategy for maintaining good water quality. Watershed management includes beneficial management practices (BMPs) that protect surface water supplies (dugouts, small reservoirs, rivers, or lakes) and groundwater aquifers (shallow or deep water supplies below ground) by reducing the potential for contaminants to enter the water source.
Some examples of substances that could contaminate a water source include sediment, fertilizer, pesticides, animal waste, animal pharmaceuticals, fuel, oil, and hazardous products used in farming or by-products used in agricultural processing.
There are three general types of beneficial practices that help reduce the risks of water quality degradation:
1. Managing Agricultural Inputs: Proper handling and use of fertilizers, manures, and pesticides will prevent or limit the impact on the environment. Nutrient management is the practice of applying fertilizers and manures only in amounts that can be taken up by the crop. Over-application increases the risk of contaminating surface and groundwater supplies.
The use of pesticides can be minimized through “Integrated Pest Management,” which includes an understanding of the target pest and the use of a combination of physical, chemical, biological, and cultural controls. Proper storage, mixing, and handling are also essential in minimizing risk to the environment.
2. Controlling Erosion and Runoff: Practices such as strip-cropping, shelterbelts, and the use of cover crops prevent erosion and reduce the movement of nutrients and pesticides from agricultural land.
Residue management through conservation tillage and continuous cropping is also effective at controlling erosion but requires higher inputs of fertilizer and herbicides.
3. Barriers and Buffers: Barriers and buffers can be planted to intercept potential contaminants from agricultural lands. In most cases, these are strips of vegetation that slow the velocity of runoff water enough for sediment to settle out, water to infiltrate into the ground, and nutrients to be taken up by plants in the vegetated buffer zone.
Grassed waterways, vegetative strips, and field borders are examples of buffers that can be used in annually cropped fields. Where buffer zones surround a stream or lake, they are usually referred to as riparian buffers.
These strips capture sediment and nutrients from water moving into the waterway from surrounding agricultural lands. The vegetation also stabilizes the banks and shores from the erosive action of the waterway itself.
Read Also: The Health Benefits of Using Accent Seasoning on your Cooking
Management of Water Sources
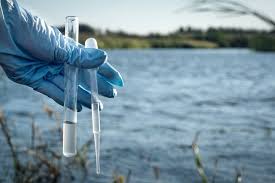
Management of the water source includes best management practices (BMPs) to sustain the best possible water quality at the source.
1. BMPs for Surface Water Sources include:
i. Proper siting and design of river or ugout intakes.
ii. Selective and limited use of algicides in farm ponds.
iii. Control of surface water inflow to the reservoir or dugout.
iv. Use of biological, chemical, or physical techniques such as aeration, coagulation, or reservoir covering.
2. BMPs for Groundwater Sources include:
i. Diverting surface water runoff away from well pits.
ii. Protecting sensitive aquifers.
iii. Preventive maintenance such as shock chlorination of wells when necessary.
Water Treatment for Use
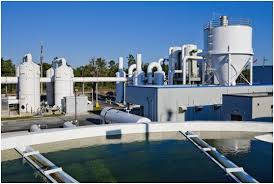
Watershed management techniques offer the first barrier for maintaining good water quality. Management of the water source is the second barrier, aimed at maintaining and improving water quality.
Recirculating systems are the most recent technology in treating wastewater from fish ponds while at the same time re-using it for the purpose of producing fish. Although both strategies will improve the quality of the source water, they cannot drastically improve water quality when pure water is necessary, such as for boilers used in the agricultural industry.
Watershed management and water source protection strategies must be combined with other treatment processes, such as in-house treatment and disinfection, to ensure that the water is safe for drinking and aesthetically pleasing for general household use.
Do you have any questions, suggestions, or contributions? If so, please feel free to use the comment box below to share your thoughts. We also encourage you to kindly share this information with others who might benefit from it. Since we can’t reach everyone at once, we truly appreciate your help in spreading the word. Thank you so much for your support and for sharing!
Read Also: A Child’s Guide to Castle Grayskall Toys: The Perfect Gift for Your Little Knight or Princess