Plant breeders have changed the genetic composition of crop species to a large degree as they selected for pest resistance and also for resistance to environmental stresses.
Such changes are in addition to the major phenotypic changes (e.g., non-shattering, uniform and fast germination) that were a consequence of domestication.
What have been the consequences of such alterations, either on the crop species and it’s near relatives or on the ecosystems in which those species are grown?
Experienced plant breeders have addressed this question as they responded to four queries sent to them. The questions were:
Have the resistance traits been stable over time?
Have they led to undesirable consequences with respect to weediness of the crop or its relatives?
What have been the major sources of pest resistance genes as used in classical breeding (e.g., same species, related species, and mutation)?
Are there relevant differences between the resistance genes currently being engineered into plants and those that have been transferred by conventional breeding?
The summary of the responses from the breeders are stated below:
Have Resistance Traits Been Stable Over Time?
The breeders say that as a general rule, resistance traits governed by major dominant genes have not been stable over time, whereas those governed by several genes have been more durable. But there are exceptions to both statements.
One cannot say categorically that single gene resistance will always be undependable, or that multiple factor resistance will always be durable.
It is important to remember that the phrase “stability of resistance” refers to whether or not a previously resistant variety is overcome by a particular species of disease or insect. It does not infer that individual resistance genes lose their power to hold individual pest biotypes in check.
The resistance genes are stable, but new (or previously undetected) pest biotypes appear, with types of virulence that are not curbed by the now-outdated resistance genes.
The variety succumbs to the disease or insect pest once again, albeit to a new race of the pest, and breeders say that the variety’s resistance was unstable.
Has Introduction of Conventional Resistance Genes Led to Undesirable Consequences with Respect to Weediness of the Crop or Its Relatives?
The breeders know of no undesirable consequences (such as enhanced competitive ability in a related weed species following the unintended transfer of resistance genes from crop to weed) from any introduction of resistance genes into crop plants through classical breeding.
Some of the introduced genes have come from very distant relatives, but all have been derived from plants. Chances of introgression from crop species to wild relatives vary by crop.
Ease of hybridization and the genetic complexity of transformation from wild to domesticated plant type (or vice versa) are major determinants for the rate and amount of introgression that might be expected.
In the US, sunflower and sorghum are highly cross-compatible with related weeds and would be the most likely crops to exhibit undesired movement of pest resistance genes from crop to weed. Breeders, however, have not yet observed this kind of introgression.
What Are the Major Sources of Resistance Genes in Classical Breeding?
The breeders say that resistance genes from within the crop species are preferred when they can be found, because of ease of breeding with them, but they will go far afield if they have to.
The practice varies with the crop; e.g., tomato breeders commonly use genes from wild relatives whereas sorghum breeders do not.
The amount of genetic diversity within the crop species and its ease of breeding with alien species are major determinants of breeders’ actions.
Are There Important Differences Between Classical and Engineered Resistance Genes?
The breeders say that engineered resistance genes now in use appear to have different modes of action than traditional resistance genes, but they point out that we know very little about structure and mode of operation of the traditional genes and so have little basis for sweeping judgments about difference.
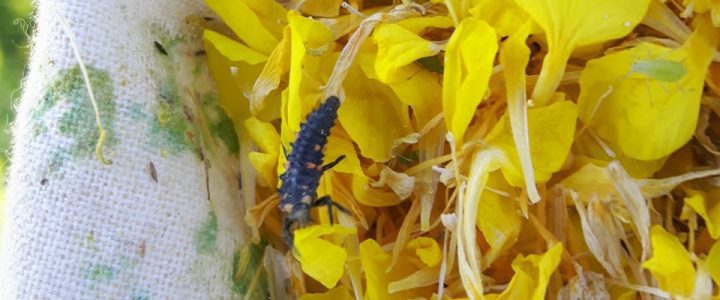
Further, we have few specifics about how a radically different genetic background might affect expression of a transgene.
Genes for herbicide resistance (the archetype example of potentially dangerous genetic transformation) are not necessarily imparted by means of genetic transformation. Such genes are found within crop species or their relatives, or have been created by means of mutation.
These genes, bred into a specific crop variety, theoretically could move from the crop to cross-compatible weed species and impart unwanted herbicide resistance to the weeds. But in order to cause a new problem, resistance genes would have to introgress into weeds that had not contributed the resistance genes in the first place.
This example shows how difficult it can be to decide whether or not a given resistance gene in a crop plant will increase competitiveness in weeds or make crop plants into weeds. Presence or absence of genetic engineering is not the major determining factor.
The breeders look to a future generation of engineered plant genes that will provide greater diversity and utility than genes presently available in any one crop.
Read Also: Plant Breeding For Disease and Pest Resistance and Their Inheritance
Genes from related taxa, from very distant taxa, or from within the crop species may be altered to provide improved resistance, but they will be plant genes rather than genes from extremely different organisms. It may be difficult to identify the point at which such new genes should be called “unnatural.”
Until recently, plant breeders did not worry about how their breeding affected weeds, or whether their crops could become weeds.
Weeds were looked on as potential sources of genes for pest resistance if they could hybridize with crop species, but almost no one thought about whether or not the population genetics of weeds could be altered by introgression from crop species.
A very few students of crop evolution studied the weeds that may have been ancestors of cultivated plants. Plant taxonomists and ecologists usually ignored weeds because they weren’t considered as parts of natural ecosystems.
Genetic engineering has changed all of that. If genes from far afield can be added to crop plants, giving them marvelous gains in pest resistance, tolerance of environmental stress, or enhanced seed production, one can imagine that those transgenes could enhance the power of weeds in the same ways.
The analogy may not be as simple as it sounds, however. Two concepts must be clarified and data need to be assembled before one can make firm predictions.
Do crop plants as a class have the same requirements for survival and luxuriance as weeds as a class?
To consider this question one must lay out the ways in which crop plants and weeds are similar and ways in which they differ.
Perhaps even before that, one must decide whether it is possible to make a definitive description of crop plants as a class, and another one for weeds as a class.
What is the functional role of resistance genes in weeds as compared to their role in crop plants?
Will a gene that greatly enhances survival chances for a crop plant perform the same service for a weed? (Crops grow in crowded monocultures; weeds usually grow in dispersed “polycultures.”)
Will the presence or absence of genetic diversity within a crop or weed population, or among crop or weed species in a site, affect the utility of a given resistance gene? (Crop varieties usually are genetically uniform, weed populations are not.)
Should one distinguish between dangers of imparting genes for resistance to natural restraints, such as disease or insect attack, and resistance to man-made restraints,such as herbicides?
Do we have any reason to believe that selection for new (or previously undetected) kinds of herbicide resistance in weed species operates on different principles than selection for new (or previously undetected) kinds of virulence in disease or insect species?
The breeders, in answering the above four questions, were considering these two main points and the subsequent questions that they raise. It is possible that they did not want to classify resistance genes into only two categories – natural or engineered.
Further, the breeders said we know so little about the molecular nature of resistance genes that we cannot yet categorize them in any meaningful way. It is possible they do not believe that mode of transfer or kingdom of origin is a meaningful classification.
Despite their reluctance to sort genes into “engineered-bad” and “natural-good,” the breeders acknowledged that whenever we fool around with Mother Nature we get surprises, some of them bad.
Therefore we need to look with caution at any novel breeding technology, predicting possible consequences as well as we can, with the modicum of data we may have in hand.
We need to know more about the effects of genetic background on gene action. Location within a genome seems important, and the entire genetic background seems important. We have little or no understanding of these interactions.
We need to know more about the consequences of hybridization of crop species with related weeds and the potential for introgression in both directions.
Jointed goat grass hybridizes with common wheat and viable backcross offspring can be produced.
Have resistance genes from wheat moved into jointed goat grass and changed its survival potential?
A similar question can be asked for sorghum and shatter cane, sunflower and wild sunflower, canola and mustards, or maize and teosinte.
So we must ask ourselves, do we have data to answer either of these key questions – effect of genetic background, or consequences of hybridization or at the least do we have enough data to let us speculate from a firmer foundation than we have at present?
In my opinion, we have fragments of data for some crops and/or their weed relatives, but rarely do we have enough for firm predictions about gene introgression or about gene action in the genome or the population.
What are the consequences of adding new pest resistance genes to a wild species, either a weed or otherwise?
How plentiful and how powerful must the genes be to change the genetic balance of the wild species, make it a stronger weed, transform a non-weed into a weed, or, conversely, reduce the weed’s viability as a competing population?
How about the “function” of related weeds as a reservoir of new biotypes of pest species, disease, or insects?
Are the weeds more dangerous to crop plants when they lack resistance and so are a constant source of pest infection and infestation?
Or are they more threatening when they contain many of the same resistance genes as carried in the crop species and therefore encourage the multiplication of new pest biotypes (biotypes that are not bothered by the weeds’ resistance genes)?
The recommendation arising from these questions seems obvious. Whenever a worrisome outcome seems likely but data are too sparse for firm conclusions, scientists need to work hard to fill the void.
They need to plan the right experiments, gather the needed data, and publicize the results in both public and specialist media. And the public needs to provide the funds to support this work, since most of it will need to be done by scientists in public institutions.
Finally, sometimes the odds of a bad outcome from not doing a particular action may be much higher than the odds of a bad outcome from performing that action. Sometimes it may be better to take action with uncertain outcome than to stand still. Life always works on probabilities.
In summary, plant diseases can also be partially controlled by use of pesticides, and by cultivation practices. Plant varieties with inherent (genetically determined) disease resistance are generally the first choice for disease control.
Breeding for disease resistance has been underway since plants were first domesticated, but it requires continual effort because pathogen populations are often under natural selection for increased virulence, new pathogens are introduced to an area, cultivation methods can favor increased disease incidence over time, changes in cultivation practice can favor new diseases, and plant breeding for other traits can disrupt the disease resistance that was present in older plant varieties.
Plant breeding for disease resistance typically includes:
Identification of resistant breeding sources.
Crossing of a desirable but disease-susceptible plant variety to another variety that is a source of resistance, to generate plant populations that mix and segregate for the traits of the parents.
Growth of the breeding populations in a disease-conducive setting.
Selection of disease-resistant individuals.
Plant diseases can be controlled by selecting and breeding plant resistant species.
Read Also : Ways To Generate Income From Electronic Waste (E-Waste)