There are currently three approaches to controlling plant viruses. Pesticides are used to control the invertebrate vector. This can be environmentally damaging (see the white insecticide deposits on the rice), and many insects develop resistance to the insecticides.
The second is clean agronomic practices such as removal of sources of infection. This can be effective for perennial crops, but in others, such as attempts to control Cacao swollen shoot virus, it did not succeed.
This approach does not work for many annual crops in tropical countries where young and old crops are grown side-by-side. The third approach is the breeding of resistance into the crop; this can be either by conventional breeding or by GM. In conventional breeding, the resistance can be to either the virus or the vector.
However, the great variability of many viruses results in the plant breeder having to play “catch up” with new virus variants that break the resistance gene. This figure shows an example of this with the reaction of various genes bred into tomato against variants of Tomato mosaic virus (related to Tobacco mosaic virus).
In practice, when one resistance gene was widely deployed, a new strain of the virus arose which broke the resistance. This has led to considerable efforts in applying GM approaches to controlling plant viruses.
Read Also: Different Systems of Rearing Rabbits
Control Measures for Plant Viruses in Agriculture
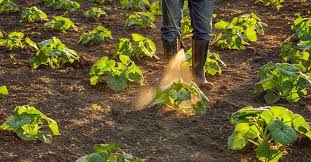
In some of the earliest experiments with transgenic plants, it was shown that the transgenic expression of some viral sequences could confer resistance to the cognate virus.
The initial experiments on transgenic virus resistance involved the expression of the viral coat protein (termed coat-protein protection); subsequently, expression of other viral gene products, either unmodified or mutated in important regions, have given some protection.
The results from some of these protein-expressing systems did not make sense, as resistance was also obtained using some non-coding sequences (the controls on the experiments).
Thus it appeared that the expressed nucleic acid was playing an important role. In some of these experiments, the expressed RNA was complementary (antisense) to the viral mRNA; in others, it was in the same sense.
This, and other observations, led to the realization that plants (and other organisms) have a previously unrecognised defence system against “foreign” nucleic acids – termed GENE SILENCING.
There are two inter-related forms of gene silencing, one that operates on the chromosomal DNA (TRANSCRIPTIONAL GENE SILENCING) and the other on the RNA in the cytoplasm (POST-TRANSCRIPTIONAL GENE SILENCING or PTGS). PTGS, also known as RNA silencing, quelling (in fungi) and RNAi (RNA interference) (in animals), has proven to be a very important feature in transgene technology.
At some stage in the replication of the “foreign” RNA, double-stranded RNA (dsRNA) is formed and the plant (or animal) recognizes this as being foreign. The right-hand part of the figure shows what happens to this dsRNA.
It is targeted by an enzyme called DICER which cuts it into pieces of 21 to 25 nucleotides (termed small interfering RNAs or SiRNAs). These then combine with various proteins to form a macromolecule termed the RNA-induced silencing complex (RISC).
Proteins in the RISC unwind the double-stranded SiRNAs which then give single-stranded SiRNAs of both polarities. The SiRNA then anneals to the target RNA (which has the same sequence as that from which it originated), making further dsRNA which is in turn targeted by DICER. Thus the “foreign” single-stranded RNA is degraded.
The importance of dsRNA in the RNA silencing process has led to constructs that transcribe to give this molecule. In this construct, the coding sequence is duplicated to give an inverted repeat, the two parts being separated by a non-coding region (intron).
This approach is opening up a whole new transgenic strategy not only against viruses but also using RNA silencing to “switch off” the expression of undesirable genes, e.g. for allergenic gene products.
There are several examples of transgenic protection against viruses in the field. These two examples were intended to involve coat protein protection, but in the light of findings on RNA silencing, there may be uncertainty as to how they operate. However, as pointed out below, it is important in making a biosafety determination to know which mechanism is involved.
Several main areas of concern have been raised about the deployment of plants transgenic in virus sequences. The main ones are: interactions with other viruses; suppression of gene silencing; spread of transgenes to other plants; possible effects on humans and other animals; stability of the transgene.
Although the transgene viral sequence will give protection against the donor virus (and possibly closely-related viruses), it will not do so against unrelated superinfecting viruses. Thus there is potential for at least three types of interaction between the transgene viral sequence and the superinfecting virus:
Firstly, as the coat protein of a virus can determine the specific interactions with the vector (insect or nematode), the concern is that the transgenically expressed coat protein can encapsidate (termed heteroencapsidate) the genome of the superinfecting virus, thus changing the transmission properties of that virus.
This happens occasionally with natural double infections of non-transgenic plants, but there is no evidence that it is more prevalent with transgenic plants.
Secondly, some combinations of viruses cause more severe symptoms than the sum of those of the two original viruses – termed synergy. The concern is that the transgene will interact with the superinfecting virus exacerbating the symptoms.
Both these concerns relate to transgenes that produce proteins. Thus, the use of the RNA silencing approach is likely to be much more efficient at protecting plants against virus infection, and so these concerns should not be important in the future.
Thirdly, the main concern about the use of viral transgenes that produce just nucleic acid is that there could be recombination between the transgene RNA and that of the superinfecting virus giving rise to a new “supervirus”. This would happen during replication of the viral nucleic acid, usually by the replicase enzyme switching strands.
It would only potentially cause a problem if the recombinant virus was viable and had a selective advantage over the superinfecting virus. There are several examples of recombination between two viruses in non-transgenic situations resulting in new viruses; one case is the devastating Uganda variant of African cassava mosaic virus that devastated the cassava crop in East Africa in the 1990s.
There are further concerns of potential hazards relating to plant viruses. The gene silencing (RNA silencing or RNA interference) phenomenon is raising two concerns about viruses and transgenic plants.
Read Also: Reasons to use Rabbit Poo (Manure) for our Soil and Crops
Firstly, as mentioned above, the CaMV 35S promoter is widely used, and infection of oilseed rape with CaMV has been shown to silence the expression of herbicide resistance expressed from that promoter.
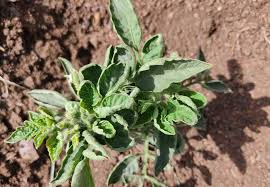
Secondly, a property of successful viruses is that as RNA silencing is a defence mechanism against “foreign” nucleic acids, successful viruses have to suppress it to be able to overcome this host defense.
Various viral genes have been identified as suppressors of RNA silencing. The question is whether this suppression of gene silencing occurs in an infection of a transgenic plant and switches off a silencing construct.
Further concerns about transgenic protection against viruses are more general and include the possibility of spread of the transgene to other plants causing effects to the environment (virus-resistant plants in the ecosystem), possible effects of the expression of viral sequences on humans and other animals (e.g. allergies), and stability of such transgenes. These will be discussed in other sections of the article.
As much is known about the detailed molecular biology, it should be possible to develop systems to minimize or obviate any hazard. Thus, viral constructs can be made that do not contain a potentially hazardous sequence, for instance, the amino acid sequences that control insect vector specificity or nucleic acid sequences that are involved in recombination. This is termed “sanitising the construct”.
However, most of these hazards are hypothetical, and the best approach is to monitor releases, a subject that will also be discussed later.
Do you have any questions, suggestions, or contributions? If so, please feel free to use the comment box below to share your thoughts. We also encourage you to kindly share this information with others who might benefit from it. Since we can’t reach everyone at once, we truly appreciate your help in spreading the word. Thank you so much for your support and for sharing!