Plant growth is a crucial process in various agricultural contexts. The ability of a plant to grow and reach a specific size within a given environment is essential for successful reproduction and achieving the desired yield. Plant growth is foundational to all agricultural productivity.
As such, it is not surprising that the processes that drive plant growth are of great importance to researchers. These processes include the biophysical and biochemical limitations on photosynthesis, ensuring respiration is not wasteful, and improving nutrient uptake and use, including water.
Researchers also focus on studying the molecular mechanisms that determine cell division. The primary goal is often to alter or optimize these processes to enhance plant growth and productivity.
Read Also: Introduction to Rabbit Rearing
Plant Environment and Light Intensity
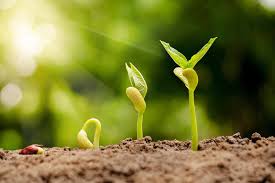
Understanding plant growth becomes more complex due to the significant influence of the environment, which can modulate different components of the growth process in various, sometimes opposing, ways.
For instance, plants grown in high light intensities tend to have higher rates of photosynthesis, resulting in a higher rate of biomass production per unit of leaf area compared to those grown in low light.
However, plants grown under high light conditions may have a reduced amount of leaf area per unit of plant mass. At light levels higher than 25 mol m−2 day−1, some species may not experience further growth stimulation despite the increased photosynthesis per unit leaf area. Physiological interactions may become more complex when multiple environmental factors interact.
Quantitative Estimates of Total Plant Mass
Plant growth models serve as simplified representations of complex systems, helping to structure and integrate available knowledge, test hypotheses, and make quantitative estimates of total plant mass, above-ground mass, and/or yield.
These models vary from simple formulas that mathematically capture plant size over time with just a few parameters to highly complex simulation models that evaluate global change effects on vegetation performance worldwide. These models often center on the plant’s carbon economy, which is the core of all growth processes.
Empirical Models of Plant Growth
Empirical models, also known as statistical models, are among the simplest models of plant mass or productivity. These models are widely used in agriculture, horticulture, and forestry to describe or forecast the productivity of monocultures of economically significant plant species.
Mechanistically, these models are considered “null models” as they do not incorporate any physiological processes. Instead, they act as dose-response curves (DRCs), relating biomass or yield observations in a given geographic location or climatic zone to a specific climatic or edaphic variable.
These models can be extended to include multiple independent factors, and regression techniques are employed to create predictive equations for biomass.
Although empirical models are straightforward and effective in predicting the productivity of natural and crop stands, they perform poorly when predicting yields outside the range of data used for their development.
For instance, crops like rice and maize are relatively cold-sensitive and thrive better at warmer temperatures. However, these models cannot accurately predict performance at excessively high night temperatures, which can cause sterility and reduce yields.
Photosynthesis–Respiration Model
A more complex approach involves models that explicitly simulate photosynthesis and respiration. These models calculate total carbon fixation in a plant or vegetation over a given time period, based on dependencies such as light, CO2, temperature, and the amount of leaf area.
After calculating the total photosynthate production, sugars required for respiration are subtracted, and the remaining sugars are distributed among various plant compartments. Ultimately, these sugars are converted to biomass, and the process repeats. This mechanism depends on:
i. The amount of nitrogen in the leaves available for the photosynthetic machinery.
ii. The extent to which the plant canopy is considered a single large leaf or multiple layers.
iii. Water or nutrient availability.
iv. Temperature constraints.
Read Also: 10 Health Benefits of Rabbit Meat
Importance of Growth Mechanism Simulations in Plants
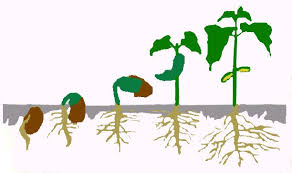
Mechanistic simulation models play a significant role in the study of plant growth. These models are valuable for predicting the growth rate or productivity of a crop in various climatic conditions.
They also provide indispensable support in management decision-making and are used to predict growth outside the strict boundaries where conditions for plant performance have been tested experimentally.
While crop modellers often accept a 10–15% difference between observed and predicted growth as reasonable, models developed for specific geographic locations tend to perform well within those regions.
Mechanistic Top-Down Models
An alternative approach to analyzing plant growth is a top-down method. This begins with the total biomass of the plant and breaks down the underlying parameters and processes into more detailed components.
This approach is often used to analyze experimental data systematically, based on carbon-economy principles. The aim is to examine which underlying processes vary between treatments, genotypes, or species, and which remain relatively constant.
Relative Growth Rate (RGR)
The most basic measure of growth is the Absolute Growth Rate (AGR), which refers to the change in plant size per unit of time. When AGR is constant, plant mass increases linearly over time.
However, AGR does not account for changes in the size of young plants, as they tend to increase biomass in a manner proportional to the biomass already present. The concept of proportional growth is captured in Relative Growth Rate (RGR), which, if constant, results in exponential growth.
Factorizing RGR into Underlying Components
By tracking both plant mass and leaf area, RGR can be broken down into two key components: Leaf Area Ratio (LAR), representing the total amount of leaf area per unit of plant mass, and Unit Leaf Rate (ULR), or Net Assimilation Rate (NAR), representing the increase in biomass per unit leaf area.
This factorization provides significant insights, as ULR is often strongly correlated with photosynthesis. By measuring plant mass and leaf area alone.
It is possible to determine whether differences in RGR are due to structural factors (LAR) or gas exchange, characterized by ULR. This approach avoids the complexities of measuring photosynthesis and respiration.
LAR can be further broken down into two components: the Leaf Mass Fraction (LMF), which is the fraction of total biomass allocated to leaves, and the Specific Leaf Area (SLA), which represents the amount of leaf area per unit of biomass invested in leaves. RGR, ULR, LAR, SLA, and LMF are the classical parameters used in growth analysis.
Although final biomass or yield serves as an indicator of how well a plant integrates various processes and organs, the primary outcome of such models is a better understanding of the intricate network of physiological and morphological traits and their response to environmental changes.
Do you have any questions, suggestions, or contributions? If so, please feel free to use the comment box below to share your thoughts. We also encourage you to kindly share this information with others who might benefit from it. Since we can’t reach everyone at once, we truly appreciate your help in spreading the word. Thank you so much for your support and for sharing!